Tunable Narrow Bandpass Fabry-Perot Filters
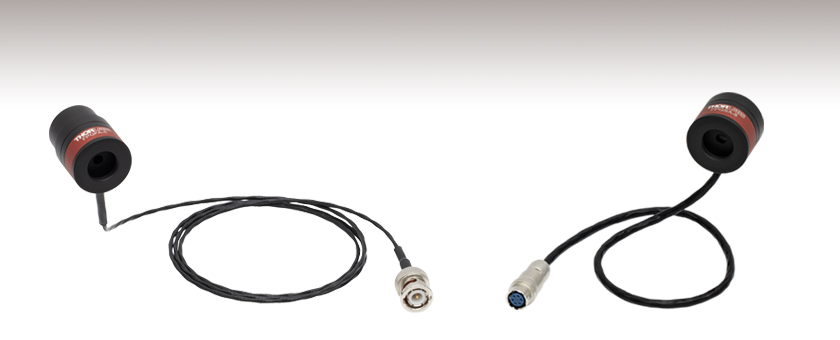
- 550 - 845 nm or 845 - 1300 nm Operating Wavelength Range
- >80% Transmission for Low-Intensity Spectra Detection
- 30 GHz Free Spectral Range
- Piezo-Tunable or Temperature-Controlled Models Available
FPQFA-5
Piezo-Tunable Fabry-Perot Filter,
Wavelength Range: 550 - 845 nm
FPQSA-8
Temperature-Controlled Fabry-Perot Filter, Wavelength Range: 845 - 1300 nm

Please Wait
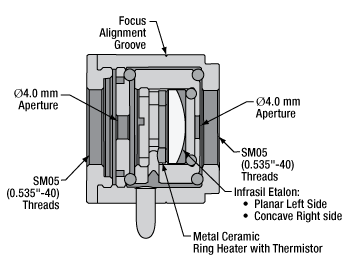
Click to Enlarge
Diagram of an FPQSA Series Fabry-Perot Filter
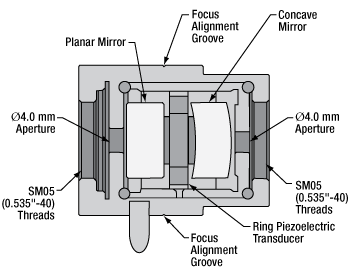
Click to Enlarge
Diagram of an FPQFA Series Fabry-Perot Filter
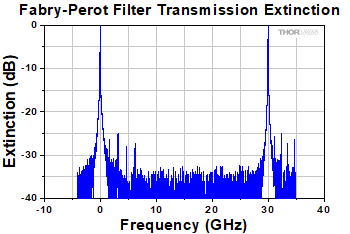
Click to Enlarge
Click Here for Data
The Fabry-Perot filter transmits fundamental resonance modes at 30 GHz FSR and suppresses off-resonance light above -30 dB (typical), not including higher order modes. The higher order modes to the right of the fundamental peaks are highly suppressed, but still transmitted in this measurement; their magnitudes depend strongly on the quality of the mode-matching.
Features
- Operating Wavelength Range from 550 nm - 845 nm or 845 nm - 1300 nm
- Filter Spectra over a Narrow Wavelength Range
- Detect Low-Light Emission from Quantum Sources
- Spectral Characterization of Quantum Emitters
- Free Spectral Range (FSR) of 30 GHz
- >80% Transmission for Low-Intensity Spectra Detection
- Finesse: >300
- Resolution: <100 MHz
- Piezo-Tunable or Temperature-Controlled Models Available
Thorlabs' FPQFA and FPQSA Series Tunable Narrow Bandpass Fabry-Perot Filters are ideal for filtering low-light emission spectra over a narrow wavelength range of about 0.1 nm (for a 1 μm wavelength laser) or free spectral range (FSR) of 30 GHz. The Fabry-Perot filters are based on a non-confocal etalon composed of two high reflectivity mirrors, one planar and one spherical concave. The FPQFA piezo-tunable filters feature a piezoelectric transducer that adjusts the etalon's mirror separation to tune the specific frequency transmitted by the etalon. The FPQSA temperature-controlled filters feature a heater attached to an etalon composed of one solid Infrasil®† 302 glass substrate that, when heated, changes its index of refraction and expands (slightly), tuning the transmission frequency. See the diagrams on the right for cross sections of each filter design.
The filters have optical coatings for wavelength ranges from 550 - 845 nm (Item #s FPQFA-5 and FPQSA-5) or 845 nm - 1300 nm (Item #s FPQFA-8 and FPQSA-8), which are typical ranges for the photoluminescence spectra of Si1 and NV2 centers in diamond and InGaAs quantum dots3. The filters' >80% transmission, 30 GHz FSR, and <100 MHz resolution allow for the investigation of the spectral fine-structures of these low-light quantum emitters. See the Specs tab for details.
Alignment
The filters' plano-concave etalon designs can achieve higher performance (optimal finesse, resolution, and transmission) with smaller mirror distances (large FSR) than a confocal etalon. However, the alignment of the plano-concave etalon is more sensitive than that of the confocal etalon as used in the SA series FP interferometers. There is a higher requirement on the matching between the illuminating mode and the fundamental mode supported by the etalon. If the mode illuminating the etalon does not match well with the fundamental etalon mode, higher order etalon modes will be excited, reducing the transmission. The illuminating mode must match in terms of mode waist size, angle, and radial and longitudinal position. Once well-aligned, the filters are capable of suppressing off-resonance light above -30 dB (typical); see the plot on the right. Follow the steps in the Mode Matching and Alignment tabs for details on aligning a beam to the Fabry-Perot filters.
Applications
The filters can be used to select a part of the incoming light's spectrum, given by the Lorentzian-shaped transmittance characteristics, and the spectrally filtered light can be used downstream in an optical system. The FPQFA series piezo-tunable filters can also be operated as scanning devices similar to the SA series FP interferometers, where the transmitted light is immediately measured using a photodiode. See the Applications tab for examples and details on the recommended controllers for use with the piezo-tunable and temperature-controlled filters.
Mounting
The Fabry-Perot filters can be mounted in one of Thorlabs' highly-stable and lockable Ø1" kinematic mirror mounts such as the
†Infrasil is a registered trademark of Heraeus Quarzglas.
References
- Lindner, S. et al., "Strongly Inhomogeneous Distribution of Spectral Properties of Silicon-Vacancy Color Centers in Nanodiamonds," New J. Phys. 20, 115002 (2018)
- Savvin, A., Dormidonov, A., Smetanina, E. et al., "NV– Diamond Laser," Nat. Commun. 12, 7118 (2021)
- Dey, A.B., Sanyal, M.K., Farrer, I. et al., "Correlating Photoluminescence and Structural Properties of Uncapped and GaAs-Capped Epitaxial InGaAs Quantum Dots," Sci. Rep. 8, 7514 (2018)
General Specifications | ||||
---|---|---|---|---|
Item # | FPQFA-5 | FPQFA-8 | FPQSA-5 | FPQSA-8 |
Wavelength Range | 550 - 845 nm | 845 - 1300 nm | 550 - 845 nm | 845 - 1300 nm |
Mirror Substrate | UV Fused Silica | Infrasil®a 302 | ||
Free Spectral Range | 30 GHz | |||
Finesse | >300 | |||
Resolution | <100 MHz | |||
On-Resonance Transmission | >80% | |||
Extinctionb | Typ. 30 dB | |||
Etalon Length | 5 mm | 3.4 mm | ||
Etalon Index of Refraction | 1 | ![]() (Click for Sellmeier Equation) |
||
Mirror Reflectance Data (Click for Graph) |
![]() Raw Data |
![]() Raw Data |
![]() Raw Data |
![]() Raw Data |
Waist and Effective Waist Datac (Click for Graph) |
![]() Raw Data |
![]() Raw Data |
||
Effective Waist Position Datac,d (Click for Graph) |
![]() Raw Data |
![]() Raw Data |
FPQFA Series Piezo Specifications | ||||
---|---|---|---|---|
Piezoelectric Transducer Voltage | 0 to 150 V | |||
Voltage for 1 FSRa | ≤22×(λ/845) V | |||
Electric Impedance | 2.2 µF |
FPQSA Series Heater Specifications | |||||
---|---|---|---|---|---|
Temperature Scanning Range | 25 to 70 °C | ||||
Temperature Scanning Range for 1 FSRa | ~15×(λ/845) - 2 °C | ||||
Heater Resistance | 50 Ω ± 10% | ||||
Thermistor Model (10 kΩ) | TDK B57230V2103F260 | ||||
Thermistor Electrical Specifications | R0 |
10 kΩ ± 1% | |||
B25/100 | 3455 K ± 1% |
FPQFA Piezo-Tunable Filter
Piezo Control Voltage (Ramp In) - BNC Male
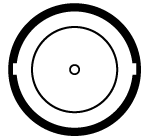
150 V Maximum
FPQSA Temperature-Controlled Filter
Female 6-Pin Hirose Connector
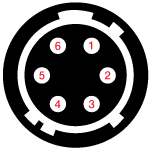
Pin | Assignment |
---|---|
1 | Heater Positive |
2 | Heater Negative |
3 | Not Connected |
4 | NTC 10 kΩ Thermistor |
5 | NTC 10 kΩ Thermistor |
6 | Not Connected |
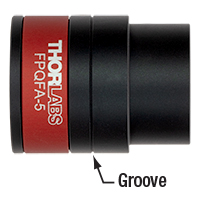
Click to Enlarge
Figure 1: The planar mirror face position in the Fabry-Perot filter is indicated by a groove on the instrument body.
Mode Matching
In order for the incident light beam to achieve resonance with the filter, the beam must be shaped to match the fundamental mode supported by the filter's etalon. The confined etalon mode is completely determined by the etalon's geometry, the wavelength, and the refractive index of the etalon substrate (either the air gapped cavity in the FPQFA filters or solid glass etalon in the FPQSA filters). To mode-match the incident beam, a lens with an appropriate focal length must be placed at the correct distance such that the beam's waist coincides with the etalon mode's waist spot size and position. The mode waist is located at the planar mirror in the FPQFA and FPQSA filters with this position indicated by the focus alignment groove on their housings, as shown for example on the FPQFA filter in Figure 1. The mode waist sizes and lens recommendations can be determined as follows:
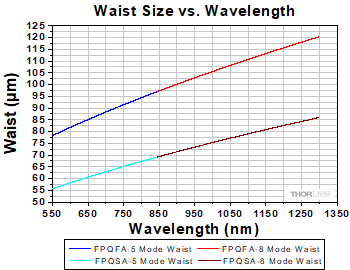
Click to Enlarge
Click Here for Data
Figure 2: The mode waist values at the planar mirror that are supported by the filter etalons are plotted from Equation (1) as a function of wavelength. Optics downstream of the filter see an effective waist size.
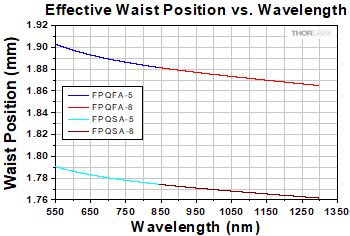
Click to Enlarge
Click Here for Data
Figure 3: Optics downstream of the filter see a shifted waist position relative to the actual waist on the planar mirror (positive values are positions after the planar mirror).
Beam Waist Calculation for Plano-Concave Etalon
The plano-concave cavity or etalon supports a fundamental mode whose beam radius or waist ωcav is on the planar mirror given by
where d, R, n(λ), and λ are the etalon length, radius of curvature of the spherical mirror, index of refraction of the etalon, and wavelength of the beam, respectively. For the FPQFA filters, d = 5 mm, R = 250 mm, and n = 1. For the FPQSA filters, d = 3.4 mm, R = 200 mm, and n depends on wavelength which can be computed using the Sellmeier equation (see the Specs tab). The waist values supported by these etalon geometries are plotted in Figure 2. Note that as the beam is transmitted through the etalon, the waist size and waist position are shifted slightly due to refraction through the spherical mirror, such that optics downstream of the filter see an effective waist size and position. The shifted positions of the effective waist after the planar mirror are plotted in Figure 3. Additional plots and data can be found in the Specs tab.
Lens Recommendations for a Free-Space Beam
A free-space beam with a measured waist ω0inc incident on a lens with focal length f will be focused to a radius
where z0inc = πw02/λ is the Rayleigh range of the Gaussian beam. The Rayleigh range is the distance from the lens the waist ω0inc of the beam has increased by a factor of √2. The lens should be placed at a distance
away from the focus alignment groove on the housing, see Figures 4 and 5 for diagrams of each etalon geometry and the lens focus distance, respectively. For z0inc >> f , Equation (3) reduces to D = f and Equation (2) reduces to
so that the ωcav values found from Equation (1) and the measured incident beam waist ω0inc at the lens determine the required focal length of the lens. For example, a collimated beam entering the lens with ω0inc = 0.56 mm and requiring ωcav = 84 µm at λ = 633 nm gives a focal length of f = 233 mm. For the same incident beam radius to mode-match an etalon waist of ωcav = 120 µm at λ = 1300 nm, a focal length of f = 162 mm is needed. Lenses with focal lengths of f = 250 mm in the first case, and f = 150 mm or 175 mm in the latter example would be reasonable choices. If the setup cannot accommodate a short focal length lens due to space constraints, increasing the incident beam waist ω0inc will also increase the required focal length. Example values for each type of filter are tabulated below.
Item # | Wavelength | Incident Waist | Mode Waist | Focal Length |
---|---|---|---|---|
FPQFA-5 | 633 nm | 0.56 mm | 84 µm | 233 mm |
FPQFA-8 | 1300 nm | 120 µm | 162 mm | |
FPQSA-5 | 633 nm | 60 µm | 167 mm | |
FPQSA-8 | 1300 nm | 86 µm | 116 mm |
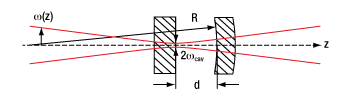
Click to Enlarge
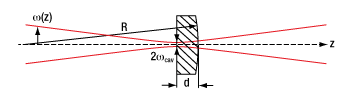
Click to Enlarge
Figure 4: Diagram of the FPQFA (top) and FPQSA (bottom) filter's etalon geometry which determines the fundamental mode-shape of the beam with waist ωcav aligned to the planar mirror.
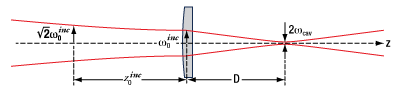
Click to Enlarge
Figure 5: Schematic showing the beam waist ω0inc incident on the lens focusing the beam to the supported ωcav beam waist at a distance D.
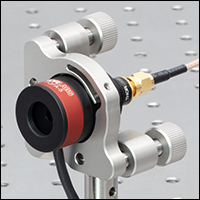
Click to Enlarge
The filters can be mounted in standard Ø1" kinematic mounts like the POLARIS-K1E shown here. An SM05-threaded mounted photodiode (Item # SM05PD2A) is attached to the filter's exit port for measurements up to 1100 nm (photodiode sold separately).
General Instructions
To get started aligning Thorlabs Fabry-Perot filters, mount the instrument in one of Thorlabs' highly stable and lockable Ø1" kinematic mirror mounts such as the POLARIS-K1E or KS1 mount or secure the filter in a fixed cage system mount such as the CP35(/M) 30 mm cage plate for Ø1" optics. Next, choose a detector compatible with the particular wavelength range and application. The Fabry-Perot filters do not include additional accessories such as a detector, allowing flexibility to customize the use of the filters according to the application. Several detector options are available from Thorlabs such as the SM05PD series mounted photodiodes, which can be attached to the filter's SM05-threaded exit port, or external detectors such as the DET series biased detectors, PDA series amplified detectors, or the APD series avalanche detectors.
Then select a lens (discussed in the Mode Matching tab) to focus the beam into the filter's etalon. After selecting the proper lens to mode-match the shape of the incident light with the filter's etalon, it is necessary to have enough degrees of freedom to optimize the alignment of the focused beam into the Fabry-Perot filter and to ensure that the waist of the beam is aligned with the planar mirror's surface. The planar mirror's position is indicated by the focus alignment groove on the housing; it is helpful to mount the filter such that the alignment groove is flush with the face of the mount, as shown in the photo to the right.
In one possible setup, the Fabry-Perot filter is secured in a kinematic mount and therefore only requires one steering mirror for alignment into the filter's etalon. The steering mirror in this case controls the horizontal and vertical position of the beam in the etalon, while adjusting the tip-tilt of the filter mostly affects the beam's direction in the etalon. This setup is recommended when a detector or camera is placed immediately after the filter, since the transmitted beam will change direction during alignment. The axial position of the beam's waist is adjusted by moving a focusing lens so that the waist coincides with the focus alignment groove of the filter's housing. The photo below on the left shows a setup with a kinematically mounted filter.
In another setup, the Fabry-Perot filter is secured in a fixed mount and therefore requires two steering mirrors for alignment. Since both mirrors affect both the position and angle of the beam, they must be optimized in pairs, as described below in the Optimizing Alignment section. As with the previous setup, the axial position of the waist is adjusted by moving a focusing lens placed before or after the mirrors, depending on the focal length and space constraints. This setup is useful when the transmitted beam will be used with downstream optics, since the direction of light is set by the fixed etalon. See the photo below on the right for an example of a setup with a filter in a fixed mount.
In either setup, an optional beamsplitter placed upstream of the lens may also be used to help establish the correct lens position. Once the waist is correctly positioned at the planar mirror, the reflected beam will be collimated, and this can be evaluated by looking at the reflection from the beamsplitter. Additionally, an aperture positioned upstream of the mirrors may be used to ensure the beam is perpendicular to the planar etalon mirror when the reflected beam from the etalon passes back through the aperture.
Two example setups to align a free-space collimated beam are shown below. The setup on the left shows an FPQFA filter in a kinematic mount, while the setup on the right shows the FPQSA filter in a fixed cage system mount.
Example Setup to Couple a Collimated Beam into a Fabry-Perot Filter
The Fabry-Perot Filters can be aligned using setups which consist of 30 mm cage system and dovetail compatible mounts secured on two 66 mm rails:
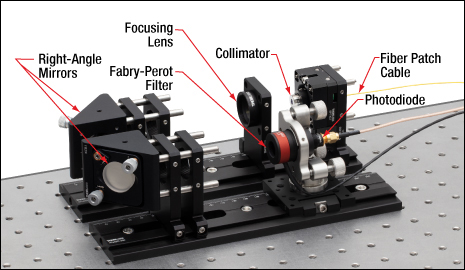
Click to Enlarge
The FPQFA-5 filter in this setup is mounted in the POLARIS-K1E mount, which provides stability and controls the angle at which the beam passes through the etalon. When this setup is aligned, the SM05PD2A photodiode, attached to the filter, will detect the beam's transmission.
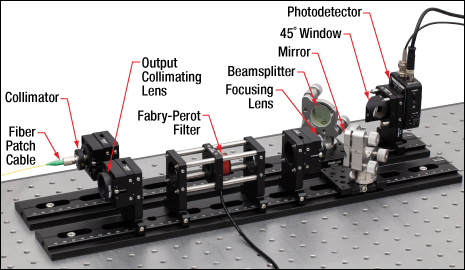
Click to Enlarge
The FPQSA-5 filter in this setup is held in a 30 mm cage system by the CP35 cage plate mount. When this setup is aligned, the transmitted beam is recollimated by the final lens for further use downstream. Additionally, a portion of the incident beam is sent to the PDA36A2 photodetector through a BSX11 beamsplitter to monitor laser fluctuations. A
The photos above show setups to mode-match a beam exiting an optical fiber to the Fabry-Perot filter. The beam exiting the optical fiber will quickly diverge and requires a collimating lens, such as the
Optimizing Alignment
For initially roughly aligning the beam, the optics should be set at the same height as the beam. It is ideal to place the mirrors with their faces at a 45° angle for 90° beam deflections from each mirror. Once the beam is centered on the filter's aperture, place the lens at the appropriate position ensuring the beam is centered on the lens to minimize deflection. Check that the beam is still centered on all the optics and adjust accordingly. The SM05A7 alignment disk can be attached to the SM05-threaded entry port of the filter to initially help center the beam.
If using the FPQFA piezo-tunable filter, connect the photodiode or detector output to an oscilloscope and turn on the piezo ramp signal, such as the output from the SA201B Fabry-Perot controller box or signal generator and amplifier, and start scanning the length of the etalon with the piezoelectric actuator. Set the amplitude at a high enough voltage to ensure that more than one peak is displayed; see section 4.5 of the FPQFA manual for details.
If using the FPQSA temperature-controlled filter, connect the photodiode or detector output to an oscilloscope and scan the frequency of the laser itself (e.g. modulate the laser drive current with a sawtooth wave) until a resonance peak is displayed from the transmitted signal. Note: A temperature controller, such as the TC300B, can be used to carefully adjust the resonant frequency of the FPQSA filter to calibrate the temperature difference between two resonance peaks and tune to a specific frequency; see section 4.6 in the FPQSA manual for details.
A subtle transmission signal can be observed once the etalon length is resonant with the wavelength of the light beam. When this signal is present, the alignment can be optimized to enhance the mode matching and increase the transmission signal. See the figures below for example output during alignment optimization. If no signal is detected at this point, it might be necessary to check the coarse alignment.
While scanning the etalon or laser frequency and carefully observing the signal on an oscilloscope, optimize the alignment of the two steering mirrors (or beamsplitter and mirror as in the setup above on the right) by walking the beam as follows: on the mirror furthest from the etalon, turn the horizontal axis knob a small amount in the clockwise direction, and then optimize the transmitted power by adjusting the horizontal axis on the closest mirror. If the power improved, return to the horizontal axis on the first mirror and turn it again in the clockwise direction and repeat. If the power is worse than when it started, return to the first mirror and turn the knob counterclockwise. Optimize the power again by adjusting the horizontal axis on the closest mirror and repeat. Continue until the power is optimized using only the horizontal axes. Repeat this procedure using the vertical axes. Iterate on both directions and until the transmission peaks are optimized. At this point there should be very little power coupling into the higher order modes. If the mode matching is still not satisfactory, it may be necessary to translate the lens axially or change the focal length.
The figures below show the oscilloscope display of the ramp signal trace in blue and the photodiode/detector output trace from the Fabry-Perot filter in yellow as the alignment improves the mode-matching of the beam to the etalon:
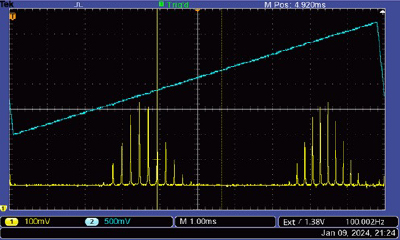
After a first iteration, the transmission improves along with many higher order modes, further optimization is required.
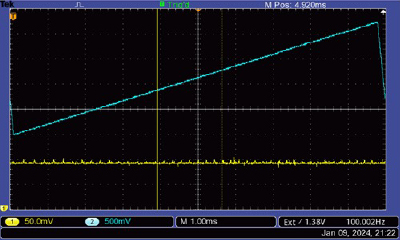
After rough alignment, many small peaks begin to emerge.
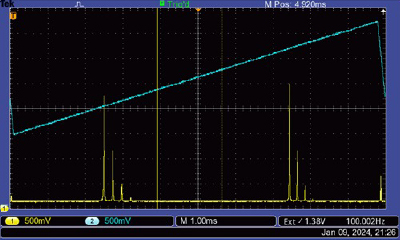
A second iteration increases the fundamental resonance mode and the higher order modes begin to suppress.
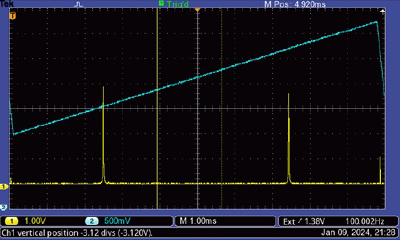
Here, mode matching is achieved and the higher order modes are mostly decoupled. Note the change in scale from 500 mV to 1.00 V of the yellow trace.
Applications Using the FPQFA Piezo-Tunable Fabry-Perot Filters
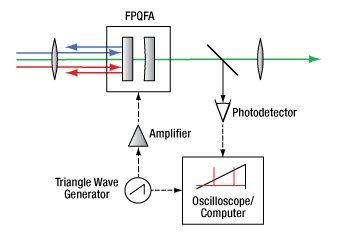
Click to Enlarge
Figure 2: Diagram of the FPQFA Filter Used to Scan Laser Frequencies
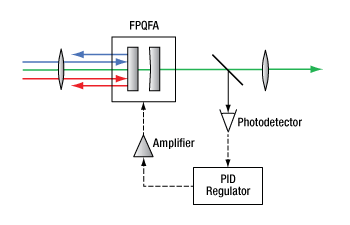
Click to Enlarge
Figure 1: Diagram of the FPQFA Filter Used in a Closed-Loop PID Feedback System
Two major modes of operation for using the FPQFA piezo-tunable Fabry-Perot filters are considered in the diagrams to the right.
Figure 1 illustrates the filters being used to select specific frequencies in a closed-loop feedback system, where the spectrally filtered light is transmitted to downstream optics for further use. Here, the transmitted resonance signal from the cavity is picked off from a beamsplitter for detection and then sent to a Proportional Integral Derivative (PID) regulator, which is amplified to continually drive the cavity back on resonance.
Figure 2 illustrates the filters being used to scan frequencies and measure the light's spectral properties. Part of the transmitted signal from the filter is immediately measured using the reflected light from a beamsplitter and sent to a photodetector, then displayed on an oscilloscope. The transmitted light that passes through the beamsplitter can be used in downstream optics depending on application. An amplified triangle wave produced by a signal generator is used to drive the filter's piezo. The signal generator's trigger signal is sent to the oscilloscope to stay in phase with the transmitted signal.
Piezo Controllers for the FPQFA Series Filters
For filtering applications, where a specific frequency is selected and locked on resonance, a high voltage amplifier, such as the MDT694B Open-Loop Piezo Controller or BPA100 High-Voltage Piezo Amplifier, combined with a signal generator may be needed to use the filters in a high-speed, closed-loop, control system. For scanning applications, which typically have lower voltage requirements, the Fabry-Perot filters can be used with the SA201B controller. This controller generates a sawtooth or triangle wave voltage, which is required to repetitively scan the length of the Fabry-Perot etalon to sweep through one FSR of the filter, and houses a transimpedance amplifier that can be used to amplify the output of a photodiode detector, which measures the intensity of the light transmitted through the etalon. The controller also provides a trigger signal to the oscilloscope, which allows the oscilloscope to easily trigger at the beginning of the scan or at a user adjustable location along the scan.
h
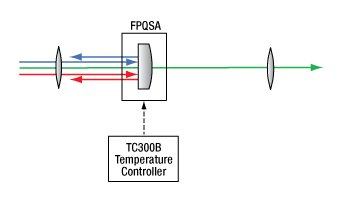
Click to Enlarge
Figure 3: Diagram of the FPQSA Filter Used with the TC300B Temperature Controller to Stabilize and Tune the Filter's Temperature
Application Using the FPQSA Temperature-Controlled Fabry-Perot Filters
Figure 3 illustrates a temperature-controlled Fabry-Perot filter being used to select specific frequencies by controlling the filter's temperature. A temperature controller with PID feedback is used to stabilize the temperature to keep the filter on resonance. After measuring the temperature change that corresponds to a 1 FSR frequency response, the transmission can be carefully tuned to a specific frequency.
Temperature Controllers for the FPQSA Series Filters
These filters are designed to sit and be stabilized at a particular resonance frequency by precisely controlling the etalon's temperature. We recommend Thorlabs TC300B Heater and TEC Temperature Controller to adjust the etalon's temperature. In order to keep the temperature constant, the TC300B controller's PID parameters in the temperature control loop must be optimized. These parameters depend on the operating temperature, environmental temperature, and variations from device to device. See section 4.6 in the FPQSA manual for the recommended procedure to set the PID parameters. Additionally, once the filter is mode matched and aligned to an incident beam, the filter's exact frequency response with respect to temperature (MHz/°C) can be measured to calibrate the temperature difference between resonance peaks. The TC300B controller can then be used to carefully tune the resonant transmission to a desired frequency. See sections 4.6.2 and 4.6.3 in the manual for additional details on calibrating and optimizing the filter's temperature response. Note: a 1 m male-to-male Hirose cable is included to adapt the FPQSA cable to the TC300B controller.
Posted Comments: | |
Ozan ARI
 (posted 2024-10-26 20:55:44.057) Hello, Can you extend the range of FPQFA-5 to 500 nm?
Can we filter laser emission to obtain clear emission spectrum using this filter? acanales
 (posted 2024-10-29 03:21:45.0) Thank you for reaching out to us! It is possible to use the FPQFA to filter out a specific wavelength. You can set the FPQFA to transmit that wavelength and use the reflected signal. The reflected signal should attenuate the chosen wavelength around 11.5 dB (OD=1.15). Note that if the signal is broader than the FSR (30 GHz), the next harmonics will also be transmitted and thus filtered from your reflected signal. I have contacted you to discuss your application and a possible customization. user
 (posted 2024-07-24 00:32:52.143) Hello, Just a quick question about the FPQFA-5 filter.
It is mentioned that this is for low light emission, so would it be appropriate for me to use it for filtering wavelengths around 738nm that are about 20Ghz apart but on the scale of single/multi photons (very low). acanales
 (posted 2024-07-25 05:43:47.0) Thank you for reaching out! The FPQFA-5 will suppress wavelengths 20 GHz from the resonant wavelength by more than -30 dB. On-resonance, more than 80% of the light will be transmitted, causing a slight decrease in the signal. Therefore, it is important to select the right detector for your single/few photons source. I have contacted you directly to discuss your application. Dong IL Lee
 (posted 2024-06-20 18:11:35.853) Hi, just for the verification;
Does the FSR of this FPQFA is still fixed (e.g 30 GHz) after tuning with the voltage? Up to my knowledge, the PZT changes the cavity length by tuning and therefore it changes FSR too. And by this way filter window can be swept around the target wavelength.
So, the tunability means the tunability of the FSR. Is this right understanding? Thank you! fnero
 (posted 2024-06-24 09:51:24.0) Hi Dong,
thanks for reaching out. Since 1 FSR corresponds to a distance of half a wavelength, it does not have any particular significance that the distance between the mirror changes, for the FSR. The manual, which can be downloaded gives more details on how this works. We have also reached out to you directly to address your questions. Ozan Arı
 (posted 2024-04-18 20:27:38.217) Can we use this device as a spectrometer to measure transmittance from a nano-cavity with 5-10 nm FSR and a few GHz FWHM, such as wavelength vs transmitted intensity with 100 MHz resolution? acanales
 (posted 2024-04-19 07:01:52.0) Thank you for contacting Thorlabs! The FPQFA is not a spectrometer but rather a very narrow bandpass filter (100 MHz) with a tunable transmitted wavelength window. It can be used to measure spectral properties like linewidth but not to obtain wavelength information. Instead, we can suggest an OSA30x with a resolution of 1.9 GHz. Unfortunately, 100 MHz is too high a resolution for our spectrometers. |

- Piezoelectric Tunable Fabry-Perot Etalon
- Compact 32.2 mm (1.27") Long Housing
- Mounts in Standard Ø1" Kinematic or Fixed Mirror Mounts
- Terminated with a 1 m Male BNC Connector Cable to Drive the Piezoelectric Transducer
The FPQFA Piezo-Tunable Fabry-Perot Filters can be used to select a part of the incoming light's spectrum for use in an optical system or to measure the spectral structures of the incoming light. The specific frequency transmitted by the etalon can be tuned by adjusting the length of the mirror separation using a piezoelectric transducer. The mirrors have optical coatings for wavelength ranges from 550 - 845 nm (Item # FPQFA-5) or 845 nm - 1300 nm (Item # FPQFA-8), which are typical ranges for the photoluminescence spectra of quantum emitters. The on-resonance transmission peaks are separated by the free spectral range (FSR) of 30 GHz, which is given by
These filters can be used to lock onto a specific resonance frequency using a high-speed closed loop feedback system or scan frequencies in order to measure the light's spectral properties. For filtering applications, a high voltage amplifier, such as the MDT694B Open-Loop Piezo Controller or BPA100 High-Voltage Piezo Amplifier, combined with a signal generator may be needed to use the filters in a high-speed, closed-loop, control system. For scanning applications, which typically have lower voltage requirements, the Fabry-Perot filters can be used with the SA201B controller. See the Applications tab for details.
Each FPQFA piezo-tunable filter has a 1 m cable terminated with a BNC connector for connection with voltage controllers and amplifiers.

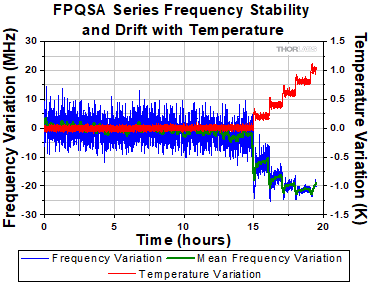
Click to Enlarge
The frequency stability of the FPQSA filter was measured at a constant temperature for about 15 hours showing <10 MHz variation. The temperature was then increased in 0.2 K steps, which correspond to the steps visible in the filter's frequency response.
- Temperature-Controlled Fabry-Perot Etalon
- Compact 22.9 mm (0.90") Long Housing
- Mounts in Standard Ø1" Kinematic or Fixed Mirror Mounts
- Terminated with a 30 cm Female 6 Pin Hirose Connector Cable (1 m Male-to-Male Adapter Cable Included)
The FPQSA Temperature-Controlled Fabry-Perot Filters can be used to select a part of the incoming light's spectrum for use in an optical system or to measure the spectral structures of the incoming light. The specific frequency transmitted by the etalon can be tuned by fine temperature control of a heater element in thermal contact with the etalon. The etalon is composed of a solid Infrasil®† 302 substrate with high reflectance coatings on the flat and curved surfaces for wavelength ranges from 550 - 845 nm (Item # FPQSA-5) or 845 nm - 1300 nm (Item # FPQSA-8), which are typical ranges for the photoluminescence spectra of quantum emitters. The on-resonance transmission peaks are separated by the free spectral range (FSR) of 30 GHz, which is given by FSR = c/2dn for the etalon length d = 3.4 mm and the index of refraction n, which is dependent on the wavelength and can be computed using the Sellmeier equation. See the Specs tab for details.
These filters are designed to be set and stabilized at a particular resonance frequency by keeping the temperature constant with a temperature controller, such as the TC300B heater and temperature controller (see Applications tab for details). In order to keep the temperature constant, the TC300B controller's PID parameters in the temperature control loop must be optimized. These parameters depend on the operating temperature, environmental temperature, and variations from device to device. See section 4.6 in the FPQSA manual for the recommended procedure to set the PID parameters. The resonant transmission can also be slowly tuned to particular frequencies by adjusting the temperature. The graph to the right shows the frequency stability and variation with temperature. It is important to operate in a temperature-controlled environment where the temperature variation does not exceed ± 0.2 °C; the performance of the device cannot be guaranteed for larger variations.
Each FPQSA temperature-controlled filter has a 30 cm cable terminated with a female 6-pin Hirose connector and includes a 1 m male-to-male Hirose adapter cable to adapt to the TC300B controller.
†Infrasil is a registered trademark of Heraeus Quarzglas.